Nuclear Fission 2.0
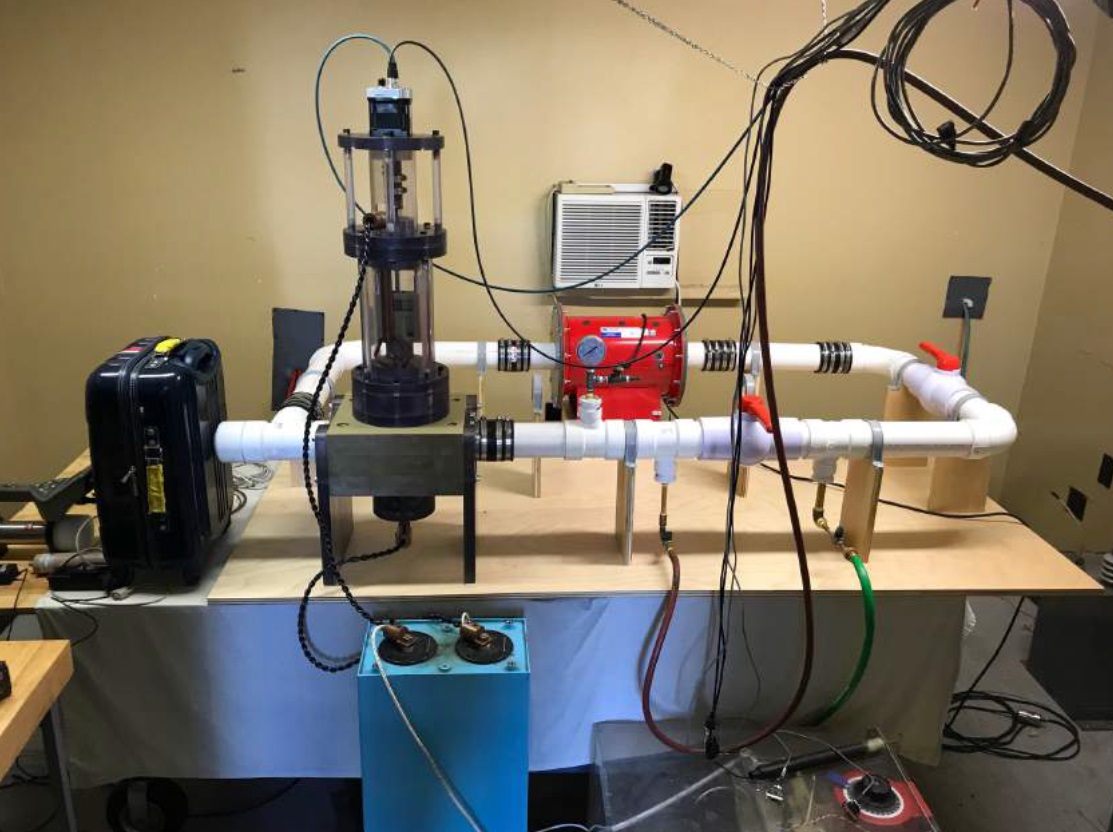
An alternative path to nuclear energy
Nuclear fission for dummies
The concept of nuclear fission and energy production is pretty straightforward:
You take a heavy atom such Uranium 235, you hit it with a neutron and watch it destabilize and break apart into 2 pieces.
During the breakup process, thermal energy is released to boil some steam and run turbines for electricity production.
Also in the breakage of said heavy atom, about 2.2 neutrons are released, of these, at least 1 (or more during power up!) must find its way back into a heavy atom of Uranium 235 and cause it to break apart to continue the chain reaction, whilst the remaining 1.2 atoms can be lost at the edge of the reactor.
Unfortunately Uranium 235 only constitutes 0.7 % of the usable isotope in natural Uranium which is made up of mostly unusable Uranium 238 at 99.3%.
Natural mineral grade Uranium must be enriched in U 235 content by means of mechanical centrifuge up to a fuel grade of about 4% U-235 to be usable inside a reactor. The nuclear fuel is considered spent and disposed of when it burns down to 1% U 235 concentration, so nuclear fuel is only used to few percentages of its total mass potential.
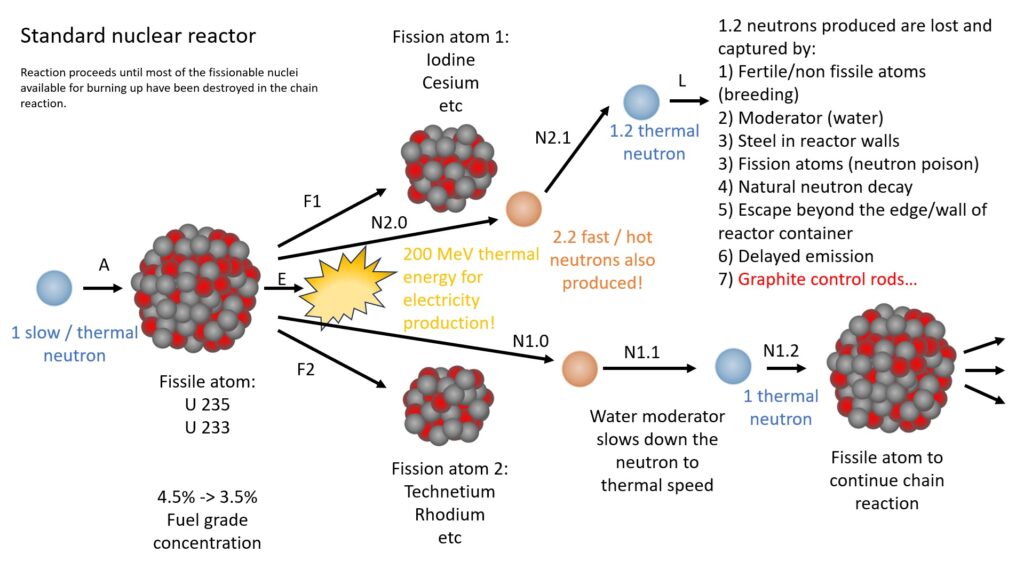
Grafite control rods are lowered or lifted to reduce or increase the number of neutrons in the chain reaction to modulate or shut down power output.
Breeding reactors
As discussed, about 1.2 neutrons are in excess and can be dispersed away from the main steady state chain reaction utilizing only Uranium 235 as the main energy source to heat up the reactor.
Of these 1.2 neutrons to spare, a certain amount will find its way and get captured by a by bystander fertile Uranium 238.
This Uranium 238 (called a “fertile” atom) then undergoes a transmutation and it changes into a fissile Plutonium 239, which can take the place of a Uranium 235 for energy production purposes.
In fact, if the reactor is neutron efficient enough to the point which, for every neutron taking away a prized Uranium 235 from the fuel, another neutron can be recycled and used to produce a fissile Uranium 239, then said reactor is called “breeding”.
In such a reactor we will be able to use the nuclear fuel to its potential 100% mass/energy, where not just all the Uranium 235 atoms will be burned up to produce energy, but also all the fertile Uranium 238 will be fully used up!
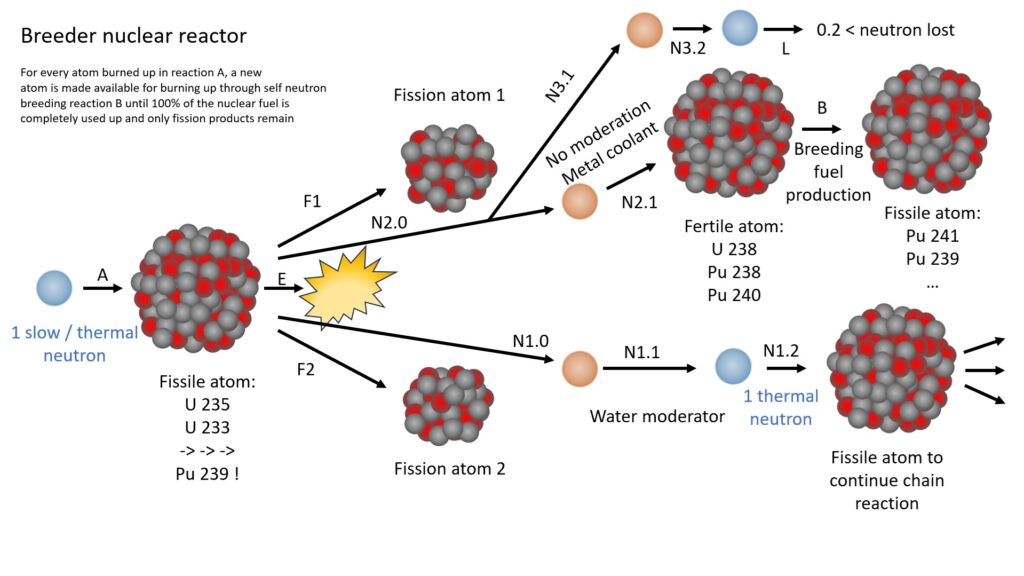
There are however very good reasons for which we do not want to use the nuclear fuel to its 100% nuclear energy potential.
Main problem with such approach is that metal of the fuel rods as well as the pressurized reactor shell undergoes neutron embrittlement because of alpha and neutron irradiation, so the metal that contains the highly radioactive fuel becomes more and more fragile and brittle as the time goes by, making it more prone to catastrophic failure with release of nuclear waste inside the reactor or in the reactor room.
Breeders also use liquid metals (potassium, mercury, lead or other low boiling temperature alloys), as a coolant instead in order to keep the neutrons fast and frisky, ideal for capturing by the Uranium 238, as opposed to the slow neutrons present in water cooled reactors, that are not good for breeding.
The liquid metal cooling and handling is much more costly and complex to operate than regular reactors running on enriched Uranium, and these reactors were eventually abandoned once it was clear that Earth was quite rich in Uranium reserves, so there was no practical need to extend the fuel efficiency of natural Uranium.
On a positive note, most of the long term residual radioactivity of spent nuclear fuel is due to leftover heavy trans uranium elements that have been breed but not burned out, whilst the low weight fission products have much faster decay and pose less of a risk in terms of long term radioactive emission.
Therefore using a nuclear fuel to its maximum potential has beneficial advantages in both reduction of total nuclear waste as well as its long term environmental hazard.
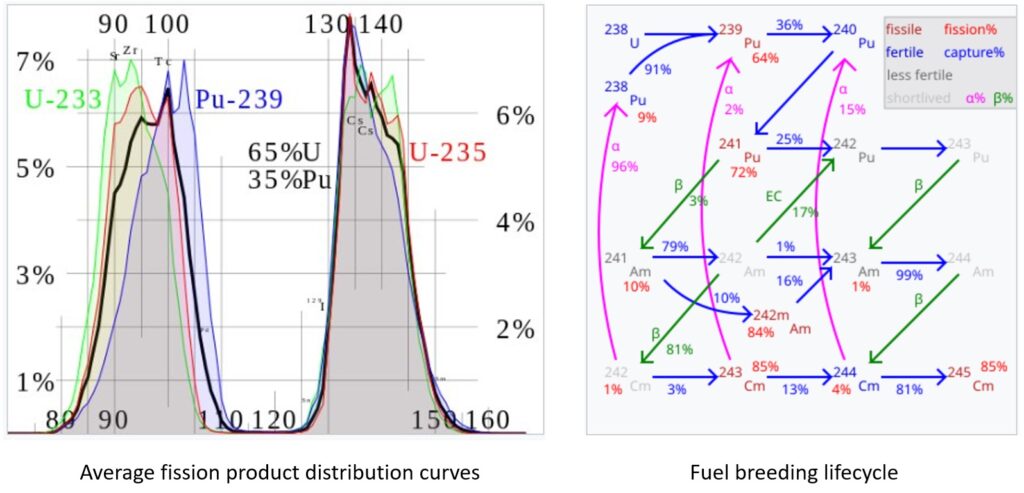
A different path to nuclear fission
As we have seen above, the neutron efficiency of a nuclear reactor and ensued geometries are critical for its correct operation as well as the use of the right moderator fluid (water, steam, liquid metal) to maintain a good fast / thermal neutron ration capable of maintaining the fission chain reaction whilst allowing also the internal self production of new fissile material within the uranium or plutonium fuel pellets.
All this is because the average neutron quantity produced in a fission event is only 2.2 so these neutrons need to be nurtured and conditioned carefully within the reactor.
On the other hand, if we were able to produce neutrons on demand, then we wouldn’t have to be so picky and snooty with reactor designs and fuel requirements and we could greatly reduce both design and operating costs whilst maintaining highest safety standards, because a low neutron efficient reactor design will swiftly shutoff (or ramp up) the fission chain reaction when the external neutron ignition source is turned off (or amped up).
The issue is however that neutrons don’t come cheap, they involve highly burdening radioactive material enrichment facilities, or very expensive particle accelerators, huge handling and disposal costs… So it appears that using the self produced neutrons from the fission event is the only way to run a commercial nuclear reactor.
Eventually some years ago, the Foundation stumbled across a very interesting piece of contemporary human technology: The directional Neutron Source (or DNS) by Thunder Energies.
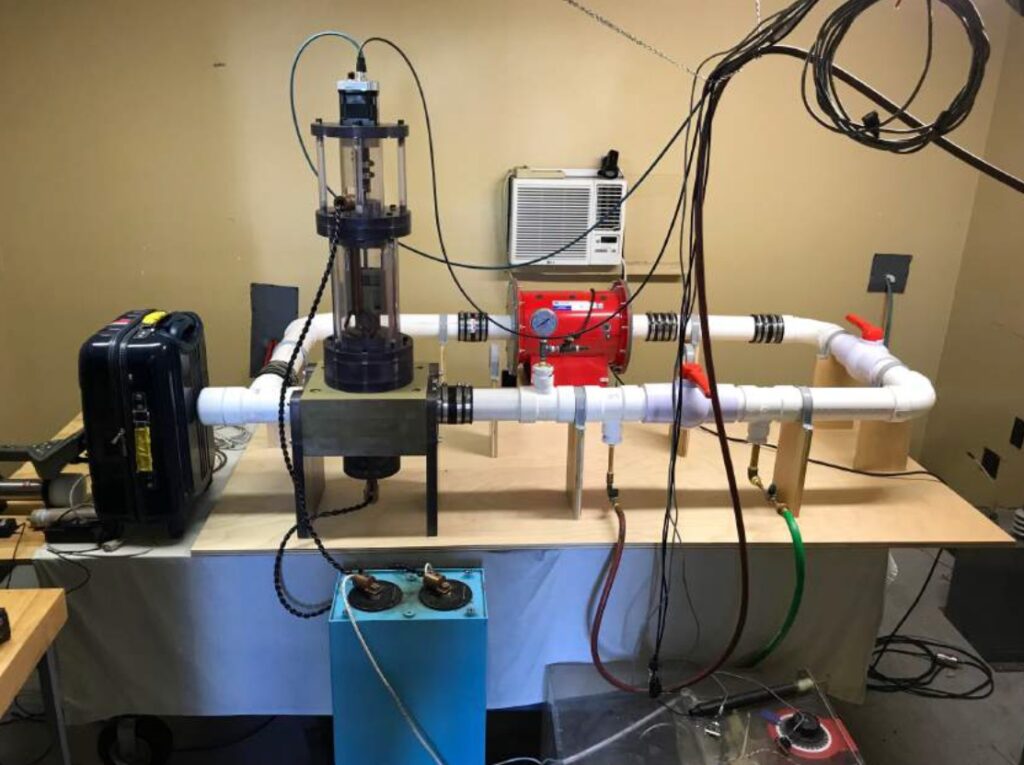
It uses a low pressure hydrogen gas and a spark tube powered by a plain 120 VAC wall plug to synthetize neutrons on the flick off a switch so to speak.
This invention went mostly unnoticed but it might have been a game changer in the nuclear industry, not just for the production of revolutionary fission reactors, but also for many other industrial applications.

(patent pending!)
One thing to be aware is the neutron production efficiency conversion (higher at higher hydrogen pressures, means intense neutron bursts which could lead to excessive fuel burn rates) to make sure we don’t spend more electricity to make the neutrons than the heat we can get to make more electricity. Still the possibility for electricity production or highly controlled and uniform burn rates for other nuclear applications is right there.
Even more interesting is the theory of operation behind this device.
Conventional particle physic wisdom has it that to make a neutron from hydrogen, like in the core of stars, it takes a great amount of pressure and temperature, and indeed all nuclear experiments in this regard focus on a HOT type approach to nuclear fusion which is quite capital and time intensive and with no commercial or economical results available up to date.
The DNS instead works the atoms smart, not hard, at low pressures and temperatures. It aligns protons and electrons onto their polar axis thanks to the strong magnetic field induced by the spark gap, and once these particles are close enough thanks to coulombian attraction, the electron subsequently “tumbles” down on the equatorial magnetic axis of the proton where it stays bond to the proton as a neutron particle.
This theory of the neutron synthesis does not need quark mutations nor neutrinos to balance out the equation in both excess mass of the produced neutrons and anomalous magnetic moment, so contemporary physicists have hard time to understand this new vista of the neutron, because they have been studying quarks and neutrino conjectures for decades and its hard to dare thinking out of the box.
If a hammer is all you have been taught, then everything will look like a quark (or neutrino) word salad.
Conventional physics explain these plasma focus machines by means of a pressure “pinch” that would cause the gas to locally reach billions of degree celsius (or about 1 MeV thermal energy) without any radiation quenching allowed before reaching said peak temperatures and it also looks the other way when challenged with the virtually null cross section of proton/electron to hit onto each other at said kinetic energies…
Bonus track: Nuclear waste 2.0
When handling nuclear waste, there are two approaches to it: 1) Concentrate and store OR 2) dilute and disperse.
The concentrate and store approach is what happens in nuclear power plants, where the spent nuclear fuel is saved inside giant pools to slowly decay over the years (or decades), notably because it is expensive to transport somewhere else and also no one wants a nuclear waste storage facility in their backyard, so once you have permitted a nuclear power plant, then you might as well use the site for short to mid term storage.
The other approach is what happens at Fukushima, where contaminated water is ultimately leaked to the open ocean for the Devil might care.
Aside from Fukushima emergency handling, the dilution solution of small quantities of waste in the middle of oceans or dispersed in the wind is both a safe and effective way of handling dangerous waste in many applications.
A third and lesser known way is to speed up and enhance the natural radioactive decay of spent nuclear fuel in matter of days as opposed to years, by means of induced decay through cyclotron irradiation.
Limited experiments have been conducted even in this worldline to date. It is up to the interested reader to find up more and follow the bread crumbs about this fascinating topic.
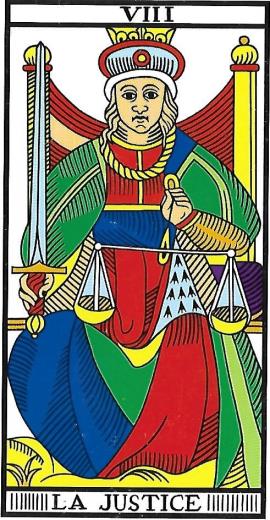